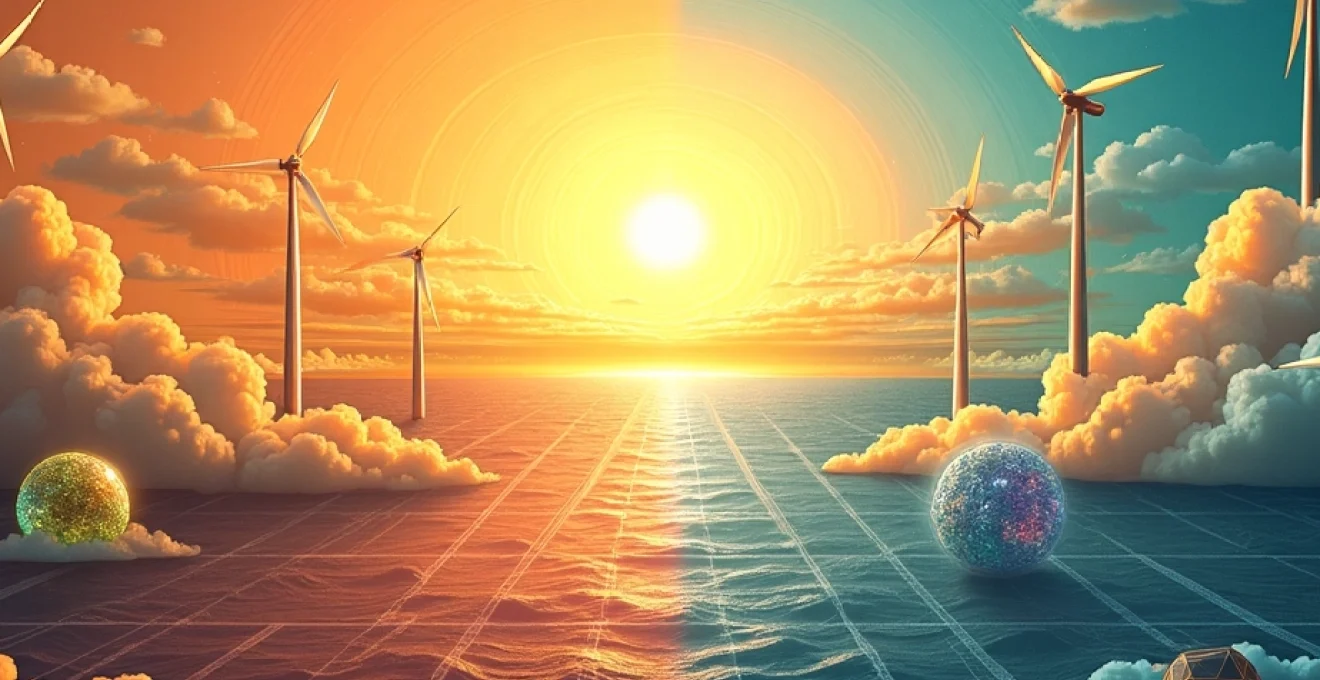
The renewable energy sector is undergoing a remarkable transformation, driven by groundbreaking innovations and technological advancements. As the world grapples with climate change and the urgent need for sustainable power sources, researchers and engineers are pushing the boundaries of what's possible in clean energy generation and storage. From next-generation solar cells to floating wind farms and revolutionary energy storage solutions, the landscape of renewables is evolving at an unprecedented pace.
Advancements in photovoltaic technology: perovskite solar cells
Perovskite solar cells have emerged as one of the most exciting developments in photovoltaic technology. These innovative cells offer the potential for higher efficiency, lower production costs, and greater flexibility compared to traditional silicon-based solar panels. The rapid progress in perovskite research has caught the attention of both the scientific community and the renewable energy industry.
Tandem Perovskite-Silicon cells: breaking efficiency barriers
One of the most promising applications of perovskite technology is in tandem cells, where a perovskite layer is combined with a conventional silicon cell. This configuration allows for the capture of a broader spectrum of light, significantly increasing the overall efficiency of the solar panel. Recent breakthroughs have pushed tandem cell efficiencies above 29%, surpassing the theoretical limit of single-junction silicon cells.
Researchers are now focusing on optimizing the interface between perovskite and silicon layers to minimize energy losses and further improve efficiency. The goal is to achieve commercially viable tandem cells with efficiencies exceeding 30%, a milestone that could revolutionize the solar energy market.
Organic-inorganic hybrid perovskites: enhancing stability and durability
While perovskites offer exceptional light-absorption properties, their long-term stability has been a significant challenge. Scientists are developing organic-inorganic hybrid perovskites to address this issue. These materials combine the high efficiency of perovskites with improved stability, potentially extending the lifespan of solar cells.
Recent studies have shown promising results in enhancing the durability of hybrid perovskites through various techniques, including:
- Incorporating hydrophobic molecules to protect against moisture degradation
- Using mixed-cation perovskites to improve thermal stability
- Developing new encapsulation methods to shield the perovskite layer from environmental factors
Lead-free perovskites: addressing environmental concerns
One of the primary concerns surrounding perovskite solar cells is the use of lead, a toxic element, in their composition. Researchers are actively exploring lead-free alternatives to make perovskite technology more environmentally friendly and commercially viable. Tin-based perovskites have shown promise as a potential substitute, although their efficiency and stability still lag behind lead-based variants.
Recent advancements in bismuth and antimony-based perovskites have also yielded encouraging results. These materials offer a non-toxic alternative while maintaining high light-absorption properties. As research progresses, lead-free perovskites could pave the way for widespread adoption of this technology in the solar energy market.
Large-scale perovskite manufacturing: overcoming scalability challenges
While perovskite solar cells have shown impressive performance in laboratory settings, scaling up production for commercial applications remains a significant hurdle. Researchers and industry partners are working on developing cost-effective, large-scale manufacturing processes that maintain the high efficiency and quality of small-scale perovskite cells.
Innovative techniques such as roll-to-roll printing and spray coating are being explored to enable mass production of perovskite solar panels. These methods could potentially reduce manufacturing costs and increase production speed, making perovskite technology more competitive with traditional silicon solar cells in the global market.
Next-generation wind energy systems
Wind energy has been a cornerstone of the renewable energy revolution, but traditional wind turbines have limitations in terms of location and efficiency. The next generation of wind energy systems aims to overcome these challenges, opening up new possibilities for harnessing wind power in diverse environments.
Floating offshore wind farms
Floating offshore wind farms represent a significant leap forward in wind energy technology. These innovative systems can be deployed in deep waters, where wind resources are often stronger and more consistent. The Hywind Scotland project, the world's first floating wind farm, has demonstrated the viability of this technology on a commercial scale.
The Hywind Scotland project consists of five 6 MW turbines anchored to the seabed using innovative mooring systems. Since its commissioning in 2017, the project has consistently outperformed expectations, achieving a capacity factor of over 50% - significantly higher than the average for conventional offshore wind farms. This success has paved the way for larger floating wind projects worldwide, with several countries now planning gigawatt-scale deployments.
Airborne wind energy systems
Airborne wind energy systems offer a radical departure from traditional wind turbines, aiming to harness stronger and more consistent winds at higher altitudes. One of the most notable examples of this technology is Makani's energy kite, which uses a tethered aircraft to generate electricity.
The energy kite operates by flying in large circles, with rotors on the wings spinning as the kite moves through the air. This motion drives generators to produce electricity, which is then transmitted down the tether to the ground. While Makani's project was discontinued in 2020, the technology demonstrated the potential of airborne wind energy and has inspired further research and development in this field.
Vertical Axis Wind Turbines (VAWTs)
Vertical Axis Wind Turbines (VAWTs) are gaining attention for their potential in urban environments and areas with less consistent wind patterns. Unlike traditional horizontal-axis turbines, VAWTs can capture wind from any direction without needing to reorient themselves, making them ideal for turbulent urban wind conditions.
Recent advancements in VAWT design have focused on improving efficiency and reducing noise, addressing two of the main challenges facing this technology. Innovations include:
- Helical blade designs that reduce turbulence and increase energy capture
- Magnetic levitation systems to minimize friction and improve low-wind performance
- Modular designs that allow for easier installation and maintenance in urban settings
These developments are making VAWTs an increasingly viable option for distributed wind energy generation in cities and built-up areas, complementing larger wind farms in rural and offshore locations.
Hydrogen economy: advancements in production and storage
Hydrogen is emerging as a crucial element in the transition to a clean energy future, offering a versatile energy carrier that can decarbonize sectors such as transportation, industry, and heating. Recent advancements in hydrogen production and storage technologies are accelerating the development of a hydrogen-based economy.
Green hydrogen: electrolysis powered by renewable sources
Green hydrogen, produced through electrolysis powered by renewable energy sources, is at the forefront of clean hydrogen production. The falling costs of renewable electricity and improvements in electrolyzer technology are making green hydrogen increasingly competitive with fossil fuel-derived hydrogen.
Key developments in green hydrogen production include:
- Advanced polymer electrolyte membrane (PEM) electrolyzers with higher efficiency and durability
- Large-scale alkaline electrolyzers optimized for variable renewable energy inputs
- Emerging solid oxide electrolysis cell (SOEC) technology for high-temperature electrolysis
These innovations are driving down the cost of green hydrogen production, with projections suggesting it could become cost-competitive with grey hydrogen (produced from natural gas) in many regions by 2030.
Solid-state hydrogen storage: metal hydrides and MOFs
Efficient hydrogen storage is crucial for the widespread adoption of hydrogen as an energy carrier. Solid-state storage technologies, such as metal hydrides and metal-organic frameworks (MOFs), offer promising alternatives to compressed or liquefied hydrogen storage.
Metal hydrides can store hydrogen at high densities and low pressures, making them potentially safer and more compact than traditional storage methods. Recent research has focused on developing new alloys with improved storage capacity and faster kinetics for hydrogen absorption and release.
MOFs, on the other hand, are highly porous materials that can adsorb large quantities of hydrogen. Their modular structure allows for precise tuning of pore size and chemistry to optimize hydrogen storage capacity. Researchers are exploring ways to enhance the stability and storage density of MOFs, with some materials showing potential for room-temperature hydrogen storage at moderate pressures.
Hydrogen fuel cells: developments in PEM and SOFC technologies
Fuel cells play a crucial role in converting hydrogen into electricity, with applications ranging from vehicles to stationary power generation. Two key technologies leading the way are Proton Exchange Membrane (PEM) fuel cells and Solid Oxide Fuel Cells (SOFCs).
PEM fuel cells are well-suited for transportation applications due to their quick start-up times and high power density. Recent advancements have focused on reducing platinum catalyst loading, improving durability, and increasing power output. These developments are making PEM fuel cells more cost-effective and competitive with battery electric vehicles for long-range and heavy-duty applications.
SOFCs, operating at high temperatures, offer higher electrical efficiency and fuel flexibility compared to PEM fuel cells. They are particularly promising for stationary power generation and combined heat and power applications. Recent research has aimed at lowering operating temperatures to reduce material costs and improve long-term stability, with some designs now capable of efficient operation below 600°C.
Energy storage breakthroughs
As the share of variable renewable energy sources in the power grid increases, efficient and cost-effective energy storage solutions become increasingly critical. Recent breakthroughs in various storage technologies are paving the way for a more flexible and resilient energy system.
Flow batteries: vanadium redox and zinc-bromine systems
Flow batteries are gaining attention for their ability to provide long-duration energy storage at grid scale. These systems store energy in liquid electrolytes, allowing for easy scaling of energy capacity independent of power output. Two prominent types of flow batteries are vanadium redox flow batteries (VRFB) and zinc-bromine flow batteries.
VRFBs offer long cycle life and the ability to fully discharge without degradation. Recent advancements have focused on improving energy density and reducing costs through the development of new electrolyte formulations and membrane materials. Some companies are now deploying megawatt-scale VRFB systems for grid applications.
Zinc-bromine flow batteries, while less mature than VRFBs, offer potentially lower costs due to the abundance of zinc. Recent research has aimed at improving the stability of the zinc electrodes and reducing self-discharge rates. These advancements could make zinc-bromine systems competitive for both stationary storage and electric vehicle applications.
Solid-state batteries: developments from QuantumScape and Toyota
Solid-state batteries represent a potential game-changer in energy storage, offering higher energy density, faster charging, and improved safety compared to conventional lithium-ion batteries. Companies are at the forefront of solid-state battery development, with promising results in recent years.
QuantumScape has reported significant progress in developing a lithium-metal solid-state battery that can charge to 80% capacity in just 15 minutes. The company's technology uses a ceramic separator to enable the use of a lithium metal anode, potentially doubling the energy density of current lithium-ion batteries.
Toyota, in collaboration with Panasonic, is working on a solid-state battery using a sulfide electrolyte. The company aims to introduce this technology in electric vehicles by 2025, promising longer range and faster charging times. These advancements could revolutionize both the electric vehicle market and stationary energy storage applications.
Thermal energy storage: molten salt and phase change materials
Thermal energy storage systems are gaining importance, particularly for concentrating solar power (CSP) plants and industrial heat applications. Molten salt storage has been widely adopted in CSP plants, allowing for electricity generation even when the sun isn't shining.
Recent research has focused on developing new salt mixtures with lower melting points and higher thermal stability, enabling more efficient and cost-effective storage. Some projects are exploring the use of liquid metals, such as sodium, as an alternative to molten salts, offering potentially higher energy density and lower costs.
Mechanical storage: advanced compressed air and flywheel systems
Mechanical energy storage systems, such as compressed air energy storage (CAES) and flywheels, are evolving to meet the needs of a renewable-powered grid. Advanced CAES systems are being developed to overcome the limitations of traditional CAES plants, which rely on underground caverns.
Isothermal CAES technology, which maintains a constant temperature during compression and expansion, promises higher efficiency and the ability to site plants in more locations. Companies are developing modular, above-ground CAES systems that can be deployed quickly and scaled as needed.
Flywheel energy storage is finding niche applications in grid frequency regulation and uninterruptible power supplies. Advanced flywheel designs using carbon fiber rotors and magnetic bearings can achieve higher energy densities and longer operational lifetimes. These systems offer rapid response times and high cycle life, making them ideal for short-duration, high-power applications in grid stabilization.
Smart grid technologies and demand response
The transition to a renewable energy-dominated grid requires sophisticated management systems to balance supply and demand. Smart grid technologies and demand response programs are evolving rapidly to meet this challenge, leveraging artificial intelligence, blockchain, and advanced communication systems.
Ai-driven grid management: machine learning for load forecasting
Artificial intelligence and machine learning are revolutionizing grid management by enabling more accurate load forecasting and optimized resource allocation. Advanced algorithms can analyze vast amounts of data from smart meters, weather forecasts, and historical usage patterns to predict energy demand with unprecedented accuracy.
These AI-driven systems can:
- Optimize the dispatch of renewable energy resources
- Predict and mitigate potential grid instabilities
- Enable more efficient demand response programs
- Reduce the need for costly peaker plants
By improving the overall efficiency of the grid, AI-driven management systems are playing a crucial role in integrating higher levels of variable renewable energy while maintaining grid stability and reliability.
Blockchain in energy trading: peer-to-peer electricity markets
Blockchain technology is enabling new models of energy trading, including peer-to-peer (P2P) electricity markets. These decentralized platforms allow prosumers (consumers who also produce energy) to trade excess electricity directly with their neighbors or other consumers on the grid.
P2P energy trading offers several benefits:
- Increased value for distributed renewable energy generation
- Reduced strain on the central grid infrastructure
- Enhanced energy resilience through local energy markets
- Improved incentives for energy efficiency and storage adoption
Blockchain-based energy trading platforms are being piloted in several countries, with projects like Brooklyn Microgrid in New York and Power Ledger in Australia demonstrating the potential of this technology. As regulatory frameworks evolve to accommodate these new models, P2P energy trading could become a significant feature of future smart grids.
Vehicle-to-grid (V2G) integration: EV fleets as grid assets
Vehicle-to-Grid (V2G) technology is emerging as a powerful tool for grid balancing and energy storage. By enabling electric vehicles to feed electricity back into the grid when parked, V2G systems can provide valuable flexibility services and help integrate higher levels of renewable energy.
Key developments in V2G technology include:
- Bidirectional charging systems that allow seamless power flow between vehicles and the grid
- Smart charging algorithms that optimize charging and discharging based on grid conditions and user preferences
- Aggregation platforms that enable EV fleets to participate in energy markets as virtual power plants
Several pilot projects are demonstrating the potential of V2G technology. For example, a partnership between Nissan, E.ON, and Imperial College London has shown that V2G systems could save the UK power grid up to £885 million annually while reducing carbon emissions.
As the number of electric vehicles on the road continues to grow, V2G integration could play a crucial role in supporting grid stability and maximizing the utilization of renewable energy resources. The challenge now lies in scaling up these technologies and developing appropriate market structures to incentivize EV owners to participate in grid services.