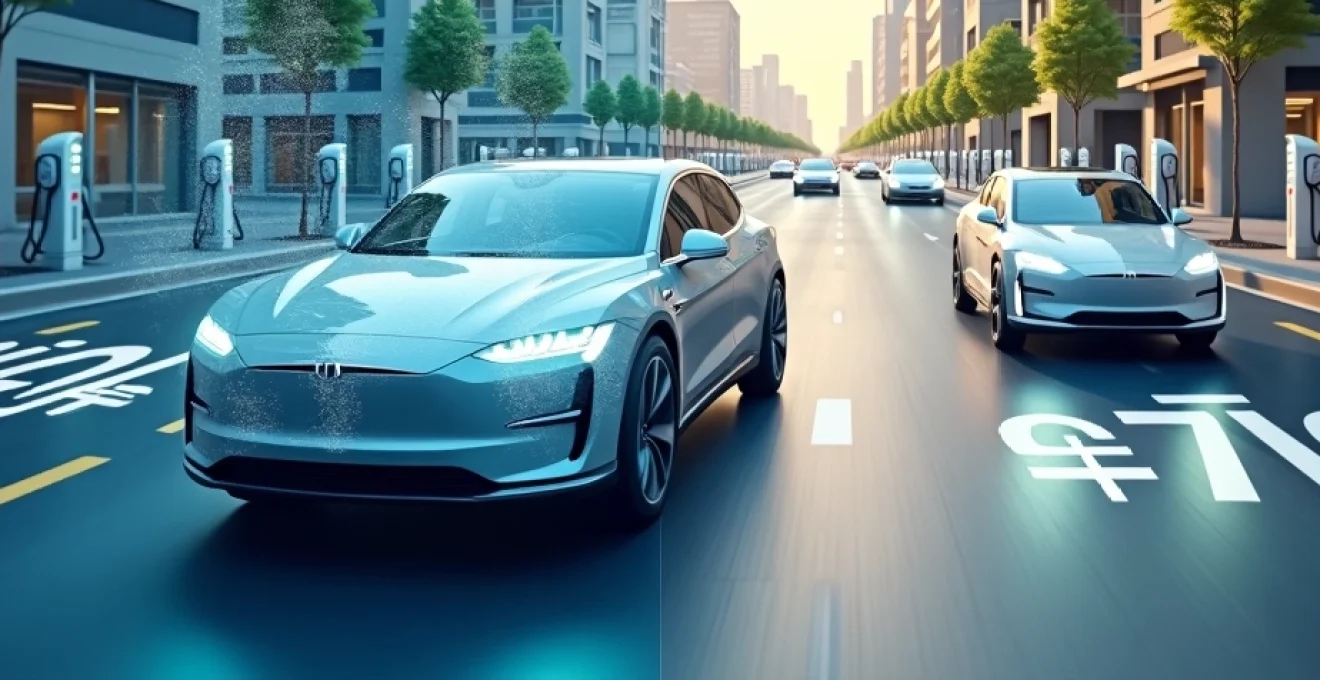
The transportation sector is undergoing a profound transformation as electric mobility gains momentum worldwide. This shift towards electric vehicles (EVs) is driven by pressing environmental concerns, technological advancements, and evolving consumer preferences. As cities grapple with air pollution and nations strive to meet climate goals, electric mobility emerges as a key solution for sustainable transportation.
Electric vehicle powertrain technologies
At the heart of every electric vehicle lies its powertrain, a sophisticated system that converts stored electrical energy into mechanical power. Unlike traditional internal combustion engines, EV powertrains offer superior efficiency, with some models achieving over 90% energy conversion rates. This remarkable efficiency translates to lower operating costs and reduced environmental impact.
Modern EV powertrains typically consist of three main components: the electric motor, the power electronics, and the battery pack. Electric motors come in various types, with permanent magnet synchronous motors (PMSMs) and induction motors being the most common. PMSMs offer higher efficiency and power density but rely on rare earth elements, while induction motors are more cost-effective and robust.
Power electronics, including inverters and converters, play a crucial role in managing the flow of electricity between the battery and the motor. These components ensure smooth acceleration, regenerative braking, and overall system efficiency. Advanced power electronics use silicon carbide (SiC) or gallium nitride (GaN) semiconductors, which offer better performance and thermal management compared to traditional silicon-based devices.
The integration of these components results in powertrains that not only deliver impressive performance but also contribute to the overall sustainability of electric vehicles. As technology continues to advance, we can expect even more efficient and powerful EV powertrains in the future.
Charging infrastructure and smart grid integration
The widespread adoption of electric vehicles hinges on the availability of a robust and accessible charging infrastructure. As EV sales surge, the development of charging networks is accelerating to meet the growing demand. This infrastructure expansion is not just about installing more charging stations; it's about creating a smart, integrated ecosystem that optimizes energy distribution and enhances the user experience.
DC fast charging networks: Tesla Superchargers vs. CCS standards
When it comes to rapid charging for long-distance travel, two main standards have emerged: Tesla's proprietary Supercharger network and the Combined Charging System (CCS). Tesla Superchargers, known for their high-speed charging capabilities and seamless user experience, have been a significant factor in Tesla's success. However, the CCS standard, supported by a consortium of automakers, is gaining traction as a more universal solution.
CCS chargers are becoming increasingly prevalent, with networks like Electrify America and IONITY expanding rapidly. These stations can deliver up to 350 kW of power, potentially adding hundreds of miles of range in just 15-20 minutes. The competition between these standards is driving innovation and improving the overall charging experience for EV owners.
Vehicle-to-grid (V2G) technology: bidirectional energy flow
Vehicle-to-Grid (V2G) technology represents a paradigm shift in how we think about electric vehicles and energy management. V2G enables bidirectional energy flow between EVs and the power grid, transforming vehicles into mobile energy storage units. This innovative approach offers numerous benefits for both EV owners and utility companies.
During peak demand periods, EVs equipped with V2G capability can feed electricity back into the grid, helping to stabilize the network and reduce the need for costly peaker plants. Conversely, vehicles can charge during off-peak hours when electricity is cheaper and more abundant. This symbiotic relationship between EVs and the grid has the potential to revolutionize our energy systems, making them more resilient, efficient, and sustainable.
Wireless charging systems
Wireless charging technology is poised to eliminate one of the remaining inconveniences of EV ownership: the need to physically plug in the vehicle. Companies like Qualcomm with their Halo system and WiTricity are at the forefront of this innovation. These systems use resonant magnetic induction to transfer power from a charging pad on the ground to a receiver in the vehicle, enabling effortless charging without the need for cables.
While currently less efficient than wired charging, wireless systems offer significant advantages in terms of convenience and durability. They are particularly promising for fleet applications, such as electric buses or taxis, where frequent charging is necessary. As the technology matures, we can expect to see wireless charging integrated into parking spaces, traffic lights, and even roadways, further simplifying the EV charging process.
Smart charging algorithms for load balancing
As the number of EVs on the road increases, managing the load on the electrical grid becomes increasingly complex. Smart charging algorithms play a crucial role in addressing this challenge. These sophisticated systems use real-time data on grid capacity, electricity prices, and user preferences to optimize charging schedules.
By intelligently distributing charging load across time and location, smart charging algorithms can prevent grid overloads, reduce peak demand, and lower costs for both EV owners and utility companies. Some advanced systems even incorporate machine learning to predict charging patterns and adjust accordingly, ensuring efficient use of energy resources and maximizing the integration of renewable energy sources.
Battery innovations driving EV performance
Battery technology is the linchpin of electric vehicle performance and adoption. Recent years have seen remarkable advancements in battery chemistry, energy density, and manufacturing processes, driving down costs while improving range and charging speeds. These innovations are not only enhancing the viability of EVs but also opening up new possibilities for energy storage in various applications.
Solid-state batteries: QuantumScape's breakthrough technology
Solid-state batteries represent the next frontier in energy storage technology. Companies like QuantumScape are pioneering this revolutionary approach, which promises to deliver higher energy density, faster charging times, and improved safety compared to traditional lithium-ion batteries. The key innovation lies in replacing the liquid or gel electrolyte with a solid material, eliminating many of the limitations and safety concerns associated with current battery designs.
QuantumScape's breakthrough technology claims to achieve an 80% charge in just 15 minutes while offering a higher energy density that could potentially double the range of electric vehicles. If successfully commercialized, solid-state batteries could dramatically accelerate EV adoption by addressing range anxiety and reducing charging times to levels comparable with refueling a conventional vehicle.
Lithium-ion vs. Lithium-Iron-Phosphate (LFP) chemistries
Within the realm of lithium-based batteries, two chemistries are currently dominating the EV market: traditional lithium-ion (often using nickel-manganese-cobalt or NMC cathodes) and lithium-iron-phosphate (LFP). Each chemistry offers distinct advantages and trade-offs, catering to different market segments and use cases.
Lithium-ion batteries with NMC cathodes offer higher energy density, translating to longer range and better performance. However, they are more expensive and rely on scarce materials like cobalt. LFP batteries, on the other hand, are cheaper, more durable, and safer, but have lower energy density. Many automakers, including Tesla, are increasingly adopting LFP chemistry for standard-range models and energy storage applications, while reserving NMC for high-performance and long-range vehicles.
Battery thermal management systems for longevity
Effective thermal management is crucial for maximizing battery life and performance in electric vehicles. Extreme temperatures, both hot and cold, can significantly impact battery capacity, charging speed, and overall longevity. Advanced Battery Thermal Management Systems (BTMS) employ various strategies to maintain optimal operating temperatures.
These systems may use liquid cooling, air cooling, or a combination of both to regulate battery temperature. Some innovative designs incorporate heat pumps that can both cool the battery and provide cabin heating, improving overall vehicle efficiency. By maintaining ideal temperature ranges, BTMS can extend battery life, improve charging speed, and ensure consistent performance across various climatic conditions.
Second-life applications for EV batteries in grid storage
As electric vehicles age, their batteries may no longer meet the demanding requirements of automotive use. However, these batteries often retain 70-80% of their original capacity, making them suitable for less intensive applications. This has given rise to a burgeoning industry focused on second-life applications for EV batteries, particularly in grid storage.
Repurposed EV batteries can be aggregated to create large-scale energy storage systems, providing valuable services to the electrical grid. These systems can store excess renewable energy, provide backup power during outages, and help balance grid load. By extending the useful life of EV batteries, second-life applications not only create new economic opportunities but also significantly improve the overall sustainability of electric mobility.
Autonomous driving and electric mobility synergies
The convergence of electric mobility and autonomous driving technologies is creating exciting synergies that promise to revolutionize transportation. As both fields advance rapidly, their integration offers numerous benefits in terms of efficiency, safety, and urban planning. Autonomous electric vehicles (AEVs) represent a vision of future mobility that is not only sustainable but also highly optimized and user-centric.
One of the key advantages of combining these technologies is improved energy efficiency. Autonomous systems can optimize driving patterns, acceleration, and braking to maximize range and minimize energy consumption. This is particularly beneficial for electric vehicles, where efficient energy management is crucial. Additionally, autonomous capabilities can enhance the charging experience by automatically navigating to available charging stations and optimizing charging schedules based on route planning and energy needs.
The integration of autonomous and electric technologies also opens up new possibilities for shared mobility services. Self-driving electric taxis and shuttles could provide efficient, on-demand transportation in urban areas, reducing the need for private vehicle ownership and easing congestion. These services could be seamlessly integrated with public transit systems, creating a more comprehensive and flexible transportation network.
Moreover, the combination of electric powertrains and autonomous systems simplifies vehicle design and maintenance. Electric vehicles have fewer moving parts compared to internal combustion engines, making them more reliable and easier to maintain. When coupled with autonomous technology, this could lead to highly efficient, long-lasting vehicles that require minimal downtime for servicing.
Environmental impact and lifecycle analysis of EVs
As electric vehicles gain prominence in the global automotive market, it's crucial to assess their environmental impact comprehensively. While EVs offer significant benefits in terms of reduced emissions during operation, a holistic analysis must consider the entire lifecycle of the vehicle, from production to end-of-life disposal.
Carbon footprint comparison: EVs vs. ICE vehicles
When comparing the carbon footprint of electric vehicles to internal combustion engine (ICE) vehicles, it's essential to consider both direct emissions from vehicle operation and indirect emissions from vehicle production and energy generation. While EVs produce zero tailpipe emissions, their overall environmental impact depends heavily on the source of electricity used for charging.
In regions with a high proportion of renewable energy in the grid mix, EVs offer substantial emissions reductions compared to ICE vehicles. However, in areas heavily reliant on fossil fuels for electricity generation, the advantage may be less pronounced. Nevertheless, as grids worldwide transition towards cleaner energy sources, the environmental benefits of EVs will continue to increase.
Studies have shown that even when accounting for battery production and electricity generation, EVs typically have a lower lifetime carbon footprint than comparable ICE vehicles in most regions.
Rare earth elements in EV motors: sustainability challenges
The production of electric vehicle motors, particularly those using permanent magnet synchronous motors (PMSMs), relies on rare earth elements such as neodymium and dysprosium. While these materials enable highly efficient motors, their extraction and processing can have significant environmental and social impacts. The mining of rare earth elements often involves energy-intensive processes and can result in environmental degradation and pollution.
To address these sustainability challenges, researchers and manufacturers are exploring alternative motor designs that reduce or eliminate the need for rare earth elements. These include induction motors, which are already used in some EV models, and new designs using ferrite magnets or other innovative materials. Additionally, efforts are underway to improve the recycling and recovery of rare earth elements from end-of-life products, creating a more circular economy for these critical materials.
Battery recycling technologies: redwood materials' approach
As the number of electric vehicles on the road increases, developing efficient and sustainable battery recycling technologies becomes increasingly important. Companies like Redwood Materials, founded by former Tesla CTO JB Straubel, are pioneering innovative approaches to battery recycling that aim to recover valuable materials and reduce the environmental impact of battery production.
Redwood Materials' process focuses on recovering key battery materials such as lithium, cobalt, nickel, and copper through a combination of hydrometallurgical and pyrometallurgical techniques. By creating a closed-loop system for battery materials, this approach not only reduces the need for new raw material extraction but also lowers the overall carbon footprint of battery production.
The company's goal is to create a sustainable supply chain for battery materials, where recycled content can be used in the production of new batteries. This circular economy approach has the potential to significantly reduce the environmental impact of electric vehicle batteries while also addressing concerns about the long-term availability of critical battery materials.
Policy frameworks accelerating electric mobility adoption
Government policies and regulatory frameworks play a crucial role in accelerating the adoption of electric mobility. Around the world, policymakers are implementing a variety of measures to incentivize EV purchases, support infrastructure development, and phase out internal combustion engine vehicles. These policies are shaping the future of transportation and driving the transition to a more sustainable mobility ecosystem.
One of the most effective tools for promoting EV adoption has been financial incentives. Many countries offer tax credits, rebates, or grants to reduce the upfront cost of purchasing an electric vehicle. For example, in the United States, the federal government provides a tax credit of up to $7,500 for eligible EVs, with some states offering additional incentives. The European Union has also set ambitious targets for EV adoption, with many member states offering substantial subsidies and tax breaks for electric vehicles.
In addition to purchase incentives, governments are implementing policies to support the development of charging infrastructure. This includes providing funding for public charging stations, offering incentives for home charger installation, and mandating EV-ready building codes for new constructions. Some cities are also implementing low-emission zones or congestion charges that exempt or favor electric vehicles, further encouraging their adoption in urban areas.
Regulatory measures are also playing a significant role in driving the electric mobility transition. Many countries and regions have announced plans to phase out the sale of new internal combustion engine vehicles in the coming decades. For instance, the United Kingdom plans to ban the sale of new petrol and diesel cars by 2030, while California has set a target for all new passenger vehicles to be zero-emission by 2035. These ambitious targets are sending a clear signal to automakers and consumers about the future direction of the automotive industry.